The metal powder-based processing of titanium: MIM and AM-related highlights from the PMTi2022 conference
Since the very first PM Titanium conference in Brisbane, Australia, in 2011, the PMTi series has been the essential meeting point where technical and commercial advances in the powder-based production of titanium parts are discussed. Encompassing production technologies from Metal Injection Molding to Additive Manufacturing, this year’s event was no different. As Dr Thomas Ebel reports, the technologies involved have moved significantly towards production readiness, but with the ever-present playoff of non-negotiable performance goals against the drive to reduce cost. [First published in PIM International Vol. 16 No. 4, December 2022 | 30 minute read | View on Issuu | Download PDF]
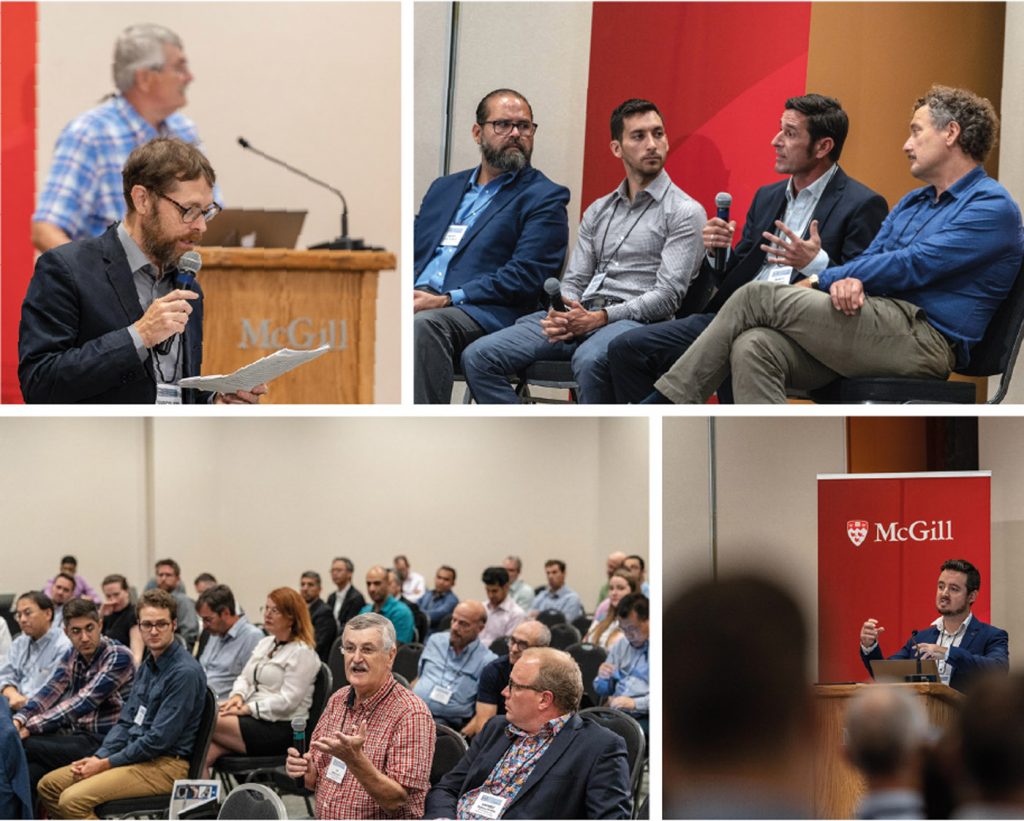
After six successful PMTi conferences, one might consider the event’s evolution by comparing the first meeting in 2011 in Brisbane and the most recent edition, held this year at McGill University in Montréal, Canada, from August 29–31. The conference was chaired by Louis-Philippe Lefebvre, National Research Council Canada; Mathieu Brochu, McGill University, and Vladimir Brailovski, ETS Montréal. Due to the ongoing pandemic, the meeting was organised as a hybrid online/in-person event; despite this, eighty participants attended in person. In total, 130 participants from twelve countries attended, illustrating the high level of interest in this conference.
Since its beginnings in 2011, originator Ma Qian intended the conference to bring together those in the titanium Powder Metallurgy industry to form a ‘task force’ for accelerating both research and industrial application of the market and, indeed, this is still a common goal of the attendees travelling long distances to take part. At the first conference in Brisbane, Australia, most of the presentations given were rather scientific in nature, but even a talk showing the ductile behaviour of a Ti-6Al-4V alloy processed by Metal Injection Molding resulted in high levels of interest. More attendees have joined the conference with each edition, creating the excellent platform that it is today for knowledge exchange between researchers and the industry.
Today, for the properties of sintered or melted titanium alloys to match those of wrought material is standard; even high fatigue properties are achieved. The number of shaping technologies, however, has increased significantly. Now, Additive Manufacturing is the main technology covered at the event, with the chief focus being on sinter-based techniques and Laser or Electron Beam Powder Bed Fusion (PBF-LB or -EB). The reality is that titanium MIM seems to be well established, with only smaller levels of research still necessary. At this year’s event in Montréal, Canada, talks given concerning MIM served mostly as a reference for AM techniques, and MIM itself was rarely the subject of further developments discussed.
In 2011, one topic was already at the forefront: cost reduction of titanium parts. By 2022, this goal was not only present at, but dominated the entire conference, with the phrase ‘low-cost’ present in almost all presentations. The most common cost-cutting strategy remains the use of ‘cheap’ powders in technologies running at the lowest costs possible. For mass production, this still means using MIM; for mid-range production volumes, Binder Jetting (BJT) is probably best suited and, for small volumes, other AM technologies may be applicable.
In Brisbane, one approach presented for cost reduction was the use of cheaper powders for non-critical applications with reduced demands for ductility; it appears that this approach has not been successful. Today’s demand is for low-cost powders which retain good mechanical properties, typically at least fulfilling ASTM standards requirements. This was the clear position of Zak Fang, of the University of Utah, who gave the first talk at the PMTi2022 conference [1]. At the start of his presentation on a holistic approach to low-cost titanium he stated, ‘Performance is non-negotiable.’
A holistic approach to low-cost titanium
In his presentation, Fang pointed out the importance of cost reduction at every step, from powder production to the finished component. From the reducing agent for Ti-sponge production, cost reduction can be enacted by using Mg rather than the much more expensive Na or Ca. The current processes from Ti containing ore or slag are still too complicated and expensive; a generally promising way is extracting TiO2 by alkaline roasting and solution hydrolysis followed by direct reduction to powder using Mg. The thermodynamical challenge for the reduction is that MgO is only more stable than TiO down to 2 wt.% oxygen – still too high of a level compared to typical standards.
With this in mind, Fang added hydrogen to the titanium oxide forming TiOH, thus increasing the oxygen potential (see Fig. 2), which becomes higher than that of MgO even for low oxygen levels. Mg can break up the titanium oxide and extract the oxygen, producing a porous, low-oxygen (< 2 wt.%, dissolved) containing titanium-hydride. In a second step, dissolved oxygen content is reduced further by the usage of molten salt (containing MgCl2) to values below 0.2 wt.% (typically below 0.12 wt.%). Now, the titanium hydride can be used directly or turned into titanium via a dehydriding process. Fang referred to the process as Hydrogen Assisted Magnesiothermic Reduction (HAMR). This process also works with Ca in place of Mg.
![Fig. 2 Change of the oxygen potential by addition of hydrogen [1]](http://www.pim-international.com/wp-content/uploads/sites/2/2023/08/Fig-02-13-1024x789.jpg)
The costs for HAMR are significantly lower than for conventional production using the Kroll or other processes and the HAMR process is easily scalable. It is expected that the process can be made cost equivalent to titanium sponge and – in combination with a Granulation, Sintering, Deoxygenation process (GSD) – it can be used to produce spherical powders from any raw material, including scrap. Comparisons with commercially available powders have shown equivalent properties of parts produced.
As Fang stated, this is only one step to cost reduction. To manufacture a low-price component, these powders have to be processed by cost effective processes. Here, MIM and AM techniques might be appropriate, depending on geometry and number of parts required. The drawback is that all powder-based processes have issues with respect to impurities, building defects, porosity, microstructure and, in particular, fatigue behaviour, which is strongly influenced by defects and microstructure size. The goal is to achieve the same dense microstructure that is found in wrought material. In the case of Ti-6Al-4V, fine globular or bimodal structures are the most common, while typical sintered material shows a coarse lamellar structure. Fang showed that, via the Hydrogen Sintering and Phase Transformation (HSPT) process, it is possible to create basically the same microstructure as in wrought material.
The alloy is loaded with hydrogen, leading to a phase diagram containing an eutectoid reaction point. This allows for similar changes in the microstructure by heat treatments (as is done in steel refining). In the case of (Ti-6Al-4V)-H, the utilisation of the six different phase regions facilitates the fine tuning of the microstructure via smart heat treatment. Fig. 3 displays a comparison between typical microstructures of wrought material and powders processed by HSPT. Fatigue tests proved equivalent properties. The static properties meet the requirements in ASTM B348 for wrought material.
![Fig. 3 Micrographs of wrought (a, b), HSPT treated (c-e) and vacuum sintered (f-h) Ti-6Al-4V. a) fully equiaxed; b) bi-modal; c+f) as-sintered; d+g) heat-treated and quenched; e+h) heat-treated and furnace-cooled [1]](http://www.pim-international.com/wp-content/uploads/sites/2/2023/08/Fig-03-13-1024x506.jpg)
HDH Ti-6Al-4V for Laser Beam Powder Bed Fusion
In many presentations at this year’s PMTi, Hydrogenation-Dehydrogenation (HDH) was a topic referenced either as it relates to the production or processing of low-cost powders. Muktesh Paliwal, from Kymera International, reported on usage of HDH powder in PBF-LB [2]. According to his calculations, material represents the second largest cost in the production of a component by PBF-LB, so a reduction in this respect would clearly be beneficial.
HDH powders are commonly irregular in shape, while spherical powders are preferred for Powder Bed Fusion processes in order to provide uniform spreadability. It is possible to apply plasma spheroidisation to the HDH powders to transform their shape, but this involves an additional process and, thus, higher costs. In the study presented, Ti-6Al-4V HDH powder with a d90 of 89 µm was used, and the optimal process parameters were determined by systematic variation of scan speed, laser power, hatch distance, stripe width and layer thickness. The fabricated samples were partly annealed at 704°C for 2 h, HIPed, or both. The oxygen content of the as-built parts resulted in 0.17 wt.%, thus meeting grade 5 requirements.
Fig. 4 shows the resulting microstructure. As shown, the HIP process closed the pores in the material and the annealing process removed the α’-martensite (dark black lines in the left image). After these processes, the tensile tests revealed a yield strength of 1039 MPa and a maximum strain of 16.1%. These values are equivalent to those of wrought material and PBF-LB specimens manufactured using spherical powders. At the end of his talk, Paliwal showed pictures of an electronic box and a topological optimised bracket – both intended for use in aerospace – which could be successfully manufactured from the irregular HDH powders.
![Fig. 4 Microstructure of PBF-LB processed HDH Ti-6Al-4V after annealing (left), HIP (middle) and annealing + HIP (right) [2]](http://www.pim-international.com/wp-content/uploads/sites/2/2023/08/Fig-04-13-1024x286.jpg)
Quasi-spherical unalloyed Ti powders from HDH powders by gas-solid fluidisation
Gang Chen, from the University of Science and Technology, Beijing, China, presented a study on quasi-spherical unalloyed titanium powders produced from HDH powders by gas-solid fluidisation [3]. The process is outlined schematically in Fig. 5. Chen estimated that the cost for these quasi-spherical powders is less than 50% of the cost of atomised ones.
![Fig. 5 Production of quasi-spherical Ti powder by gas-solid fluidisation [3]](http://www.pim-international.com/wp-content/uploads/sites/2/2023/08/Fig-05-13-1024x532.jpg)
Depending on processing temperature and time, the d50 value can be fine-tuned between about 28–34 µm due to interparticle adhesion, which also allows adjustments in flowability. Processing at 450°C for 10 min led to a Hall flowability below 40 sec/50 g, comparable to those of typical plasma and gas atomised powders. The oxygen content was determined to be 0.16 wt.%, compared to 0.13 wt.% of the raw powder. The apparent density was increased from 2.04 to 2.48 g/cm3. To evaluate the suitability of powders for Additive Manufacturing, three different PBF-LB machines were used to produce different shaped specimens and parts successfully. The tensile properties of the as-built Ti parts using the fluidised HDH powders were comparable to those of more expensive atomised powders.
Improving densities of titanium alloys using the CHAD method
At PMTi 2019, Chengshang Zhou, from Central South University, Changsha, China, showed that hydrogen treatments can be used for the densification of powders or porous sintered parts, similar to HIP. In Montréal, he presented further promising results using the CHAD (Constrained Hydrogenation Assisted Densification) method [4].
Using the CHAD method, the powder or sintered part is enclosed in a rigid cell and subjected to heat treatment under hydrogen. The hydrogen uptake allows the part to expand, which leads to high compressive stress in its confined state. The result is a densification of the initially porous material. Fig. 6 shows the process chain of sintering, hydrogenation and dehydrogenation. It is important to ensure that the relationship between the hydrogen content and temperature is such that the material is ductile (represented by the green area in the phase diagram), meaning that the hydrogen has to be in solution and without a TiH2 phase.
![Fig. 6 Processing chain of CHAD and phase diagram of the system Ti-H [4]](http://www.pim-international.com/wp-content/uploads/sites/2/2023/08/Fig-06-12-835x1024.jpg)
In his study, Zhou looked at the effects of the CHAD process on CP-Ti and Ti-6Al-4V powders. Fig. 7 shows the microstructure of the as-sintered CP-Ti and that, after CHAD, porosity analysis by microscopy and µCT revealed the closure of most pores. The remaining ones were reduced in size and uniformly distributed, revealing the isotropic type of compressive process. Processing of Ti-6Al-4V led to a similar result. Tensile tests, in particular, showed a significant improvement in ductility. CP-Ti achieved a 451 MPa yield strength and 17% elongation after sintering at 1200°C and subsequent CHAD processing, while Ti-6Al-4V reached 959 MPa and 12%, respectively.
![Fig. 7 Microstructure of CP-Ti as-sintered (A) and after CHAD (B) [4]](http://www.pim-international.com/wp-content/uploads/sites/2/2023/08/Fig-07-11-1024x443.jpg)
The powder-based processing of TiNi alloys
At prior events, Efraín Carreño-Morelli from the University of Applied Sciences and Arts Western Switzerland has pointed out the benefits of TiH2 for sintering. At the PMTi conference in Montréal, he summarised the previous work on the processing of Ti-Ni alloys and added some more interesting information on recent successes [5]. Ti-Ni alloys are of interest because of their shape memory capability and superelastic behaviour; in the medical industry, they have already seen extensive use in components like stents.
In his talk, Carreño-Morelli presented the materials physics explanation of these special properties – basically a transformation between a martensitic and an austenitic phase by both mechanical and thermal load. He pointed out the importance of alloy composition on the efficiency of the effects and the transformation temperatures. Typically, the materials are conventionally processed in a rather elaborate way by hot and cold working, machining, and a final thermal treatment. Powder Metallurgy could reduce the costs by net-shape production and reduction of scrap, but it is difficult to purchase appropriate pre-alloyed powders, while usage of elemental powders needs careful monitoring during sintering.
In all cases, possible contamination is an issue accompanying the whole processing chain and can influence the final properties of the sintered part. According to the ASTM F2063-05 standard for wrought TiNi, the content of oxygen and nitrogen must not exceed 0.05 wt.% in total, which is a very low value with respect to sintered titanium alloys.
Carreño-Morelli evaluated previous studies on the choice of base powders used to form the alloy: Pre-alloyed powders lead to good results, but are very expensive, while blending Ni and Ti powders leads to reactions that are difficult to control. Elemental TiH2 and Ni powders, however, have appeared as a promising alternative.
The powders used had a d50 value of 9.8 µm for TiH2 and 6.9 µm for Ni carbonyl. They were blended with polyethylene, paraffin wax and stearic acid to form a PIM feedstock. The sintered density of ‘dog bone’ tensile test specimens was 97.5%, with transformation heat and peak temperatures similar to the values of comparable melt-cast alloys. The shape memory behaviour is shown in Fig. 8, where a recoverable strain of about 4% under 100 MPa tensile stress is visible, illustrating the positive results when manufacturing with the powder mixture.
![Fig. 8 Shape memory behaviour of Ni-Ti fabricated from elemental Ni and TiH2 powders by MIM [5]](http://www.pim-international.com/wp-content/uploads/sites/2/2023/08/Fig-08-9-1024x982.jpg)
In an analogous manner, the utilisation of pre-alloyed powders for PIM feedstock led to good shape memory properties, too, even if the content of oxygen was 0.12 wt.%. By improving the debinding process, carbide formation was reduced, and a heat treatment involving quenching made it possible to avoid precipitation of the Ni4Ti3 phase, which is detrimental to the material’s shape memory properties.
Carreño-Morelli also showed examples for the processing of pre-alloyed and elemental powders by SG-Additive Manufacturing (Solvent on Granules Additive Manufacturing, a PBF technique using locally applied solvents on granulated powder and binder), and Binder Jetting. In all cases, basic shape memory properties could be achieved.
The highlighted research shown was Ni-Ti as processed via PBF-LB. Here, pre-alloyed powder with d50 of 40.3 µm was successfully processed to different demonstration geometries (e.g., stents and files). In contrast to other studies, test specimens were examined under tensile load. Fig. 9 shows the very good results with respect to the superelastic properties of as-built and heat-treated material.
![Fig. 9 Superelastic behaviour of pre-alloyed Ni-Ti powder processed by PBF-LB (tensile test) [5]](http://www.pim-international.com/wp-content/uploads/sites/2/2023/08/Fig-09-8-1024x630.jpg)
Overall, the presentation showed that Ti-Ni can be processed from different powders and by various PM technologies to achieve sound properties, though costs, contamination and processing details are still important issues. Carreño-Morelli announced that more research on SG-AM and Binder Jetting is in progress. He closed his presentation noting that Ti-Ni samples made by PBF-LB will be part of an investigation about materials ageing under space conditions, which will take place within the ESA/CNES funded project Euro Material Ageing (Fig. 10).
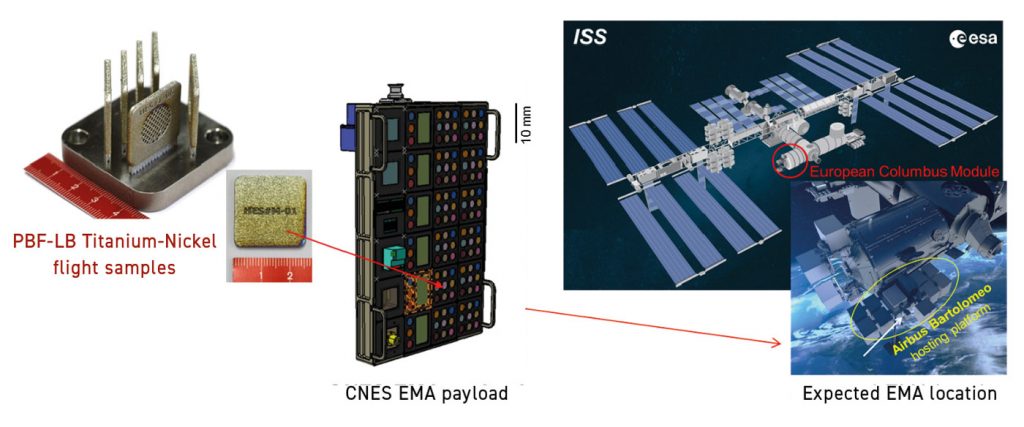
Evaluation of nitinol produced via powder-based forming routes
PM fabrication using Ni-Ti powders was also the topic of a talk by Paul Sheffields, Praxis Technology, USA [6]. As mentioned above, the processing is possible, but difficult – and not all challenges have been mastered. In particular, the shape memory effect is difficult to control – the focus of Sheffields’ presentation was on superelasticity.
The Austenite Finishing transition temperature Af must be exceeded to achieve superelasticity, but this can be difficult, as it is affected by interstitial level, Ni content, density and microstructure. As previously described, the elemental approach is connected to hardly controllable reactions between the powders, which results in unwanted phases and other drawbacks like Kirkendall porosity and eutectic melting.
Pre-alloyed gas atomised powders with a d50 of 20 µm were produced for the study and processed by PBF-LB, MIM and Binder Jetting. For PBF-LB, the powder size was 15–53 µm. In the case of the specimens made by BJT, it turned out that sintering under argon did not lead to sufficient density; sintering under a pure hydrogen atmosphere, however, worked well, and a density of 95% could be achieved. In the case of MIM – after optimisation of the sintering parameters to 1260°C / 6 h – a density of 78% was achieved in an argon atmosphere.
Processing by PBF-LB yielded the highest density, at 98%. Despite sintering in hydrogen, the interstital levels in the BJT samples (O: 0.381 wt.%; C: 0.124 wt.%) were significantly higher than in the MIM material (O: 0.17 wt.%; C: 0.036 wt.%). The hydrogen level was low in all samples.
According to different interstial content and density, the evaluation of the Af transition temperature revealed different values. While PBF-LB led to an Af of 57°C, MIM and BJT resulted in 92°C and 97°C, respectively. These values are well above room temperature, so superelastic properties must also be tested at these elevated temperatures.
A simulation of an additional HIP treatment by applying a heat treatment (1050°C / 3 h) under vacuum lowered the Af value in the case of MIM and BJT (sintered in Ar), while increasing it for PBF-LB specimens. In the latter case, this effect was possibly due to grain coarsening, but further research wasn’t able to be undertaken in this study due to geometric restrictions and testing issues. Sheffields announced his intended future work in achieving higher densification in MIM and BJT specimens, HIP processing and adjustment of the debinding step in order to reduce the interstial content. This – and a possible addition of Ni – should lower the Af transition temperature to room temperature.
Opportunities for titanium in hydrogen technologies: The case of bipolar plates
Carlos Romero, from University Carlos III in Madrid, Spain, showed that hydrogen cannot only be used for facilitating the processing of titanium, but also to produce hydrogen [7]. He reported on results gathered from the EU project PERMEABLE’s work on the production of titanium Bipolar Plates (BP) for electrolysers. These plates are part of the Proton Exchange Membrane (PEM) cells used for the separation of oxygen and hydrogen from water molecules by application of an electric field. PM production of these plates and the connected porous transport layers (PTL) could significantly reduce their costs.
Aside from the mechanical and geometrical properties, two other characteristics are essential for the performance of the BPs: corrosion resistance and interface contact resistance (ICR) between BP and PTL. Pure oxygen and hydrogen are highly reactive, thus titanium requires a coating to reduce the corrosion rate – coatings that might increase the ICR, which would be detrimental to the BP’s performances. As a solution, a nitriding process was chosen, resulting in a TiN coating. Because TiN is conductive, the ICR decreases compared to Ti alone, whilst also improving the corrosion resistance.
In the work presented by Romero, he produced porous Ti structures from TiH2 mixed with space holders from NH4HCO3. Different sizes of space holders were used, up to 500 µm, and porosity levels between 49–62% were realised. Sintering took place under argon at 1000°C for 2 h and, subsequently, a nitriding process was applied under a pressure of 1 bar of pure nitrogen at 1000°C for 2 h. This process showed that a one-step sintering and nitriding process was beneficial with regard to the achieved properties. XPS analysis of the surface revealed a composition of 31 at.% TiN and 69 at.% Ti(O,N)/TiO2. The thickness of the layer amounted to several hundreds of nm.
![Fig. 11 Effect of nitriding on the interface contact resistance ICR [7]](http://www.pim-international.com/wp-content/uploads/sites/2/2023/08/Fig-11-6-1013x1024.jpg)
Fig. 11 shows the differences in ICR depending on the treatment. Nitriding lowered the value significantly; this held true even after corrosion. While these are promising results, Romero reported that recent studies suggest the corrosion conditions in PEMEC may be less aggressive than expected. This means that coated steel might be a viable option, so it isn’t clear if the results from this study will facilitate practical application of PM of titanium in future.
Titanium MIM and Binder Jetting
Robert Swenson, from TriTech Titanium Parts, USA, compared his company’s experience with Binder Jetting with its experience of MIM [8]. Even if most of the processing chain is identical, he stated, a big difference exists in the time needed to start production. For MIM, the bottleneck is the manufacturing of the mould, which typically lasts two-to-five months before the final geometry is fixed. In BJT, however, the evaluation of a design and management of possible shrinkage and deformation from sintering only takes two-to-five days.
Swenson pointed out some of the typical differences of MIM and BJT leading to different shrinkage behaviour. In MIM, because of the high solid load of the feedstock and the isotropic injection moulding process, the powder packing of the green part is uniform and shrinkage can be assumed to be almost the same in all directions. In contrast, BJT produces a lower green density, which might lead to different shrinkage in the x, y and z directions.
Swensen presented three ways in which manufacturers can counter deformation during sintering, some of which are also used in MIM:
- Use of ceramic setters the part can land on
- ‘Live setters,’ which are auxiliary parts additively manufactured together with the component and thus shrink in the same way
- The use of a simulation software (like Desktop Metal’s Live Sinter, which is delivered together with its BJT machines). After calibrating the software to the deformation of a sample part, the geometry of the green part is adjusted with respect to the expected distortion
Table 1 compares the typical properties of Ti-6Al-4V processed by BJT and MIM. As shown, MIM achieved slightly better properties – while binder jetted material meets the requirements of the ASTM MIM standard F2885, there is still room for optimisation with respect to powder size distribution, binder and processing parameters. Which technique is the best choice depends on production volume and complexity, as well as the necessary precision, surface quality and further parameters.
![Table 1 Typical properties of specimens made from Ti-6Al-4V by MIM and BJT [8]](http://www.pim-international.com/wp-content/uploads/sites/2/2023/08/Table-01-4-1024x546.jpg)
Ti-6Al-2Sn-4Zr-2Mo alloy rods fabricated using Cold Spray, HIP and Spark Plasma Sintering
A promising technology to create larger preforms or parts with high mechanical properties in comparably short time is Cold Spray. Christian Doblin from CSIRO in Melbourne, Australia, presented a comparative study of a Ti6242 (Ti-6Al-2Sn-4Zr-2Mo-0.8Si) alloy processed by three different Powder Metallurgy techniques: Cold Spray, HIP and Spark Plasma Sintering (SPS) [9]. All results were compared to the properties of wrought material. The study was specifically geared towards reducing the production cost of net-shape preforms for high-temperature applications.
Pre-alloyed powder in a size range of 15–45 µm served as raw material for the three techniques. All specimens produced were characterised with regard to chemical and mechanical properties, as well as to porosity and microstructure. Cylinders with a diameter of almost 30 mm and a height of 85 mm were produced by CS using nitrogen gas at 900°C and 6 MPa pressure. The powder feed was 480 g/h. After Cold Spray, the rods were machined to 25 mm diameter and 76 mm length. Some rods were additionally HIPed at 900 °C, 200 MPa, for 4 h, reducing the porosity from 6.5 to 0.3%. The CS process increased the oxygen content from 0.08 to 0.17 wt.% and the nitrogen level from less than 0.01 to 0.02 wt.%; the additional HIP process did not change these values. Thus, the amount of O and N is within the corresponding specification.
SPS and HIP of the powders as alternative routes were performed within the α/β-regime of the alloy. All materials were subjected to rotary swaging as a thermal-mechanical-processing step (TMP) and to a final duplex annealing. A comparison of the microstructures revealed freedom from defects and equivalence to the wrought material. Tensile tests were performed at room temperature, at 316°C and at 538°C. All PM materials showed similar or better strength and ductility than the wrought material. Fig. 12 showcases the results for UTS and YS at 538°C.
![Fig. 12 UTS and YS of Ti-6242 specimens produced by different techniques; S: SPS; C: Cold Spray; CH: Cold Spray + HIP; H: HIP; W1 and W2: wrought material [9]](http://www.pim-international.com/wp-content/uploads/sites/2/2023/08/Fig-12-6-1024x633.jpg)
In his summary, Doblin highlighted the fact that Cold Spray proved as a promising method for net shape preform production in connection to potential cost reduction.
Cold Spray AM: CP titanium breakthroughs
This was also the thesis of Alex Finch from Titomic USA, a company focused on Cold Spray Additive Manufacturing. His presentation aimed in pointing out the benefits of this technology with respect to performance and economics [10]. Titomic’s facilities enable a build rate of up to 45 kg/h with a resultant maximum part size of about 9 x 3 x 1.5 m. Finch discussed the economic and environmental advantages of using HDH Ti powders instead of gas atomised Ti-6Al-4V for the Cold Spray process. Because HDH Ti powders are extracted directly from the Ti sponge, expensive steps preparing the raw material for gas atomisation can be avoided. Moreover, only one fourth of the energy for gas atomisation is needed in the case of HDH production.
Finch showed that mechanical properties like strength and elongation to fracture of the Cold Spray material can be tuned to the requirements of the application by oxygen content and processing parameters – for example, a UTS of 727 MPa combined with 10% elongation is one of the possible specifications which is sufficient for many applications. In his last slides, Finch showed some pictures of products produced by Cold Spray from HDH Ti powders. One example was a deployable satellite antenna hub of 60 kg weight and 1.3 m diameter, which was produced for about half the costs of conventional production from billet because of Cold Spray’s near-net shape production. Finch showcased a thin-walled propellant/chemical processing tank of about 35 cm length and 18 cm diameter (Fig. 13). The thickness is customisable. The component was built in less than one hour and no joining areas or heat affected zones – both of which could be critical for failure of the tank – were needed.
![Fig. 13 Thin wall tank, near-net shape preform manufactured by Cold Spray of HDH Ti powders [10]](http://www.pim-international.com/wp-content/uploads/sites/2/2023/08/Fig-13-5-1024x639.jpg)
Cold rolling and annealing of Ti-6Al-4V and Ti-5Al-2.5Fe roll compacted strips
While Cold Spray has been a feature of PMTi since its beginning, Shannon Clemens, from Dalhousie University, Nova Scotia, Canada, presented her work on another technique which is only rarely referenced: Direct Powder Roll (DPR) [11]. Her work on the DPR compaction of titanium alloy strips was motivated by a potential cost reduction, because the energy-intensive issue of ingot production and processing can be avoided. For her investigation, Clemens used two different powder mixtures with the compositions Ti-6Al-4V and Ti-5Al-2.5Fe, both well-known α/β-alloys. The aim was to optimise their processing with respect to density, microstructure and mechanical properties.
Roughly, the powders were roll compacted to strips, sintered, and then cold rolled. For the study, four different rolling paths were developed (as shown in Fig. 14).
![Fig. 14 Different processing chains investigated of Direct Powder Roll [11]](http://www.pim-international.com/wp-content/uploads/sites/2/2023/08/Fig-14-5-1024x494.jpg)
The evaluation of the chemical composition revealed a large scattering of Al, V and Fe content, respectively. It appears that there is a loss of these elements during processing, which cannot be distinctly allocated to the rolling path itself, though the reason for this scattering is not yet clear. The oxygen contents of the final parts were in the range between 0.25-0.36 wt.%. UTS and ductility achieved values between 570-860 MPa and from 2 to 11%, respectively. The scattered composition and oxygen levels made it difficult to make a clear correlation. Clemens was able to determine the relationships between titanium content and oxygen as well as oxygen content and UTS, though no clear effect of oxygen on ductility was visible. A general result from this study was that the DPR facilities were able to produce high-density strips using all four paths. With respect to wrought material, it was possible to achieve comparable strength and ductility.
Scaling sinter-based titanium AM technologies
Once more, PMTi2022 showed how many different technologies based on powders exist. This is especially the case when it comes to Additive Manufacturing technologies which involve binder and require debinding and sintering steps. In two talks, Matthias Scharvogel [12] and Johannes Schaper [13] from Element 22, Germany, gave an overview of some of these techniques, as well as of their commercial applications and performance.
The two presentations showed that, in general, the mechanical properties of sintered titanium alloy parts are equivalent to those of specimens manufactured by PBF-LB or PBF-EB, if adequate powders, binders and sintering processes are used. This result is basically independent of the shaping technology applied. As long as the fabrication process does not introduce specific critical defects, the sintering process results in similar properties.
Scharvogel reported on a comprehensive study using spherical Ti-6Al-4V powders under 20 µm, produced by Element 22’s ‘SBS’ sintering process, which lends itself to fine microstructures and low porosity (typically between 0.5 and 2%). While keeping powder and sintering process constant, the researchers applied a number of different shaping techniques including MIM, filament- and pellet-based Material Extrusion (MEX), Cold Metal Fusion (CMF) and Tritone’s MoldJet technology.
Filament-based MEX utilises metal-filled filaments, while pellet-based MEX is based on normal MIM feedstock granules. CMF processes granules made from a binder/metal powder mixture in a conventional polymer PBF-LB machine. MoldJet is a rather novel technique, in which a polymeric mould is built, layer by layer, each of which is filled with a paste of binder and metal powder. The technique is relatively fast, precise and particularly well-suited for larger parts. In all cases, a debinding step was added to the shaping process, followed by an identical sintering process.
As is visible in Fig. 15, MIM processing achieved the highest strength and ductility, though the AM techniques resulted in similar properties. In all cases, the specification of ASTM F1472 was exceeded significantly. Furthermore, a comparison against PBF-LB and PBF-EB revealed equivalent properties. Thus, sinter-based Additive Manufacturing of titanium alloys can be taken as a serious alternative to melting technologies based on laser and electron beam.
![Fig. 15 Comparison of UTS, YS and elongation of specimens made from Ti-6Al-4V powders by different AM technologies and MIM (* = as-built, ** = heat treated) [12]](http://www.pim-international.com/wp-content/uploads/sites/2/2023/08/Fig-15-4-1024x470.jpg)
The two presentations pointed out the importance of powder, binder, equipment and processing details when working to achieve high mechanical properties. In his talk, Schaper focused on the influence of the binder. On the one hand, this is the main source of contamination by oxygen and carbon, but, on the other, binder is needed for shaping and green part stability. Each shaping technology requires specific binder properties – in all cases, the best compromise must be found.
There are some basic rules for binders. First, the amount of binder should be as small as possible (especially during the thermal debinding step). Second, solvent and heat treatment should be as environmentally friendly (using water-based solvents) and the level of residuals as small as possible. For fulfilling the latter demand, thermoplastics are beneficial, while thermosets are more difficult to remove. Schaper emphasised the importance of knowing exactly how the polymers are composed and how their decomposition takes place. Only then can the thermal debinding step be optimised to minimise contamination.
Schaper summarised his talk in a table shown in Table 2 comparing different sinter-based technologies with respect to the kind and amount of binder used, speed of production, resolution, technological readiness level (TRL), contamination, density and properties of the sintered part.
![Table 2 Comparison of binder-based sintering techniques with respect to various aspects of processing, properties and industrial application [13]](http://www.pim-international.com/wp-content/uploads/sites/2/2023/08/Table-02-4-1024x587.jpg)
Comparing the fatigue properties of Ti-6Al-4V, processed by MIM and pellet-based MEX
A further comparison of MIM and sinter-based AM was presented by Wolfgang Limberg from Helmholtz-Zentrum Hereon, Germany [14]. His topic of choice was filament-based MEX, in this case referred to as Fused Granular Fabrication (FGF). He used plasma atomised Ti-6Al-4V powders with a size < 20 µm and produced fatigue specimens by MIM and pellet-based MEX. The motivation of the work was to determine if prototypes made by MEX can mirror the dynamic properties of parts made by MIM. If so, this would make MEX an ideal additional technique for a MIM producer because the identical feedstock granules and the same debinding and sintering equipment are used by both applications.
Limberg used a binder composed of paraffin wax, PE-EVA copolymer and stearic acid; the metal powder content was 33.5 vol.%. Solvent debinding was done in hexane, sintering was performed in vacuum at 1300°C for one hour. The fatigue samples were fabricated as 44 mm length bars and tested via a four-point bending test, R = – 0.2. To avoid crack initiation at the surface, the bars were shot peened using zirconia beads. The oxygen content of the powder was relatively high (0.21 wt.%), but, because of rather low oxygen uptake during processing, the sintered MIM and MEX specimens kept a value of 0.24 wt.% – well below the commonly accepted limit for embrittlement around 0.33 wt.%. The residual porosity of MIM and MEX material was 1.7% and 1.9%, respectively. Limberg attributed this small difference to manufacturing defects like pore strings he found between layers and between infill and perimeter. These defects also affect the fatigue properties.
Fig. 16 shows the fatigue curves of MIM and MEX fabricated samples. For comparison, the results of MIM specimens made from powder with a size < 45 µm are also included. The main difference between the curves is the high scatter of the test results of the MEX samples. Some of the specimens were equivalent to the MIM fabricated ones, while others show significantly lower fatigue resistance. This is a typical sign of irregularly occurring defects of different locations, geometries and sizes. In addition to the defects between layers, Limberg identified connected pores at the surface, also originating from a non-optimal build process. As a result of this study, one can conclude that the material itself is equivalent to MIM material, but the manufacturing quality has to be optimised in order to achieve reliable properties – a statement true for all AM technologies.
![Fig. 16 Fatigue properties of Ti-6Al-4V produced by MIM and MEX [14]](http://www.pim-international.com/wp-content/uploads/sites/2/2023/08/Fig-16-4-1024x686.jpg)
Assessing metal powder spreading behaviour for powder bed-based AM applications
Defects can also result from sources other than the Additive Manufacturing process itself. For example, it can be related to inhomogeneous distribution of powder particles. In Powder Bed Fusion processes, spreadability of the powders is an important issue. Spreadability can change from powder batch to batch, however, so a defined determination of this property is necessary. Salah Eddine Brika from École de technologie supérieure, Montréal, Canada, presented a running project on a novel testing bench of the spreading behaviour of powders, in particular aiming at PBF-LB machines [15].
Brika started his talk with a general description of the spreading process, which takes 30-50% of the total build time. The powder itself determines the rheological behaviour, the characteristics of the resulting powder bed and the spreading forces. Thus, the goal was both to determine the spreadability of a given powder and to develop a guideline for the selection of suitable powders. This guideline is intended to be adaptable to any given powder, if the relationships mentioned are known.
The powder bed should have maximum density, the best uniformity and spreading forces should be minimal. Brika listed a number of techniques for flowability characterisation of a powder, most of which already boast standards. While there are also established powder spreadability testers, the problem is that in-situ investigations inside of typical Additive Manufacturing machines are difficult because of their closed architecture and harsh environment. Furthermore, there is a lack of characterisation methods to determine simultaneously the aforementioned metrics of powder bed density, uniformity and spreading forces. Thus, Brika developed a versatile testing bench for assessment of these parameters, while the controlled variables are layer thickness, spreading speed, powder characteristics (e.g., size distribution and morphology) and the delivery system characteristics (type, material, etc). The proposed design of the test bench – replicating the coating elements of a PBF-LB machine – is shown in Fig. 17.
![Fig. 17 Basic design of a test bench for determination of powder spreadability [15]](http://www.pim-international.com/wp-content/uploads/sites/2/2023/08/Fig-17-3-1024x817.jpg)
In order to monitor the necessary metrics, the test bench is equipped with load cells under the build plate as a weighing system to measure the powder bed density, an imaging system to assess the powder bed uniformity and a torque sensor connected to the recoater arm to determine the spreading forces. Some details in the construction avoid influence of friction on the load cells. Determination of powder bed uniformity is done via a photometric stereo method.
Currently, the first case study is in progress, with very promising results. As an example, Fig. 18 shows the resulting powder bed density in relation to recoating speed for three different layer thicknesses. It can be seen clearly that the dependence of density on recoating speed increases with increasing layer thickness. At the end of his talk, Brika pointed out that other sub-systems could be added like a gas shielding chamber, different spreading mechanism types and blade shapes, heating units and thermal sensors, etc.
![Fig. 18 First results on powder bed density measured on the novel test bench [15]](http://www.pim-international.com/wp-content/uploads/sites/2/2023/08/Fig-18-2-1024x788.jpg)
Small batch atomisation of specialised spherical Ti-based powders
Ti-6Al-4V is well known as the most widely used titanium alloy, providing well balanced properties for most application fields. But Ti-6Al-4V, of course, isn’t appropriate in all cases (e.g., high-temperature applications). The development of novel alloys, however, is hindered by a lack of availability of pre-alloyed powders. Blending elemental powders might be an option, but often the sintering process suffers and porosity increases. Fortunately, some powder producers offer atomisation of small batches of specialised titanium alloys for specific applications.
![Fig. 19 Adjustment of the powder size distribution by EIGA processing parameters [16]](http://www.pim-international.com/wp-content/uploads/sites/2/2023/08/Fig-19-1024x522.jpg)
As one example, Karin Ratschbacher from GfE Metalle und Materialien GmbH, Germany, a subsection of AMG (Advanced Metallurgical Group), outlined the production of specialised powders for medical applications [16]. Apart from Ti-6Al-4V as a standard material, β-titanium alloys are used and under development. For these alloys Mo, Nb and Ta are typical alloying elements, making the preparation of the alloy and its atomisation more difficult because of very different melting points. Ratschbacher applied the well-known EIGA technology and, by adjustment of the atomisation process, it was possible to adjust the particle size distribution depending on the technology applied (e.g. MIM, PBF-LB, PBF-EB) as shown in Fig. 19. If the melting points of the alloying elements are not too high, specific alloys can be produced using Induction Skull Melting (ISM) for casting of smaller rods as shown in Fig. 20. For titanium combined with components of higher melting points – like Ti-Mo-Zr-Fe – powder can be produced through a Powder Metallurgical feedstock production route or via a three-step process involving electron beam melting, ISM and EIGA.
![Fig. 20 Application of Induction Skull Melting to fabricate rods for EIGA [16]](http://www.pim-international.com/wp-content/uploads/sites/2/2023/08/Fig-20-1024x361.jpg)
Fatigue behaviour and failure mechanisms of MIM beta titanium alloys
β-titanium alloys are especially interesting for biomedical applications because they can consist of exclusively biocompatible alloying elements like Ti, Nb and Zr. In contrast, Al and V in the Ti-6Al-4V are known to induce possible negative effects on the human body. A study on a comparison between β-titanium alloys and α/β-alloys with respect to the effect of defects on the mechanical properties of MIM produced specimens was presented by the author of this review [17].
At the beginning of my talk, I emphasised the importance of fatigue behaviour, as it is estimated that fatigue accounts for 90% of mechanical engineering failures. Past work on the effect of porosity and contamination by oxygen and carbon on the fatigue resistance of α/β alloys like Ti-6Al-4V and Ti-6Al-7Nb was presented. In this alloy class, a clear decrease of fatigue endurance limit with increasing oxygen content can be observed, while residual porosity plays a minor role compared to grain size. Grain refinement improves the endurance limit significantly in Ti-6Al-4V. The question of the study presented in Montréal was how β-alloys compare with these results with respect to the typical three MIM defects: residual porosity, impurities and coarse microstructure.
For this investigation, a Ti20Nb10Zr alloy was formed by blending spherical gas atomised powders and processing them via MIM. Cuboids with a size of 44 x 5 x 3 mm were produced for a four-point bending test as fatigue specimens and shot-peened with zirconia beads after sintering to avoid surface cracks. I pointed out that more than 90% of the fatigue lifespan is taken up at the crack initiation stage, while crack propagation is comparably fast. Thus, the goal of development of a fatigue resistant titanium alloy is to hinder crack initiation.
Microstructural investigations revealed that in β-titanium alloys porosity appears to play the major role for crack initiation, in contrast to the findings in α/β-alloys. On the other hand, oxygen appears beneficial: in β-titanium alloys oxygen increases the strength without significantly deteriorating the ductility. The role of carbon remained unclear within the study that I reported on. Because of rather low carbon solubility of the β phase, the Ti-20Nb-10Zr formed carbides; a high amount of carbides can lead to a bimodal behaviour of the fatigue curves at high stress levels (Fig. 21), connected to a possible fracture of the carbides. The endurance limit, however, appeared not to be influenced.
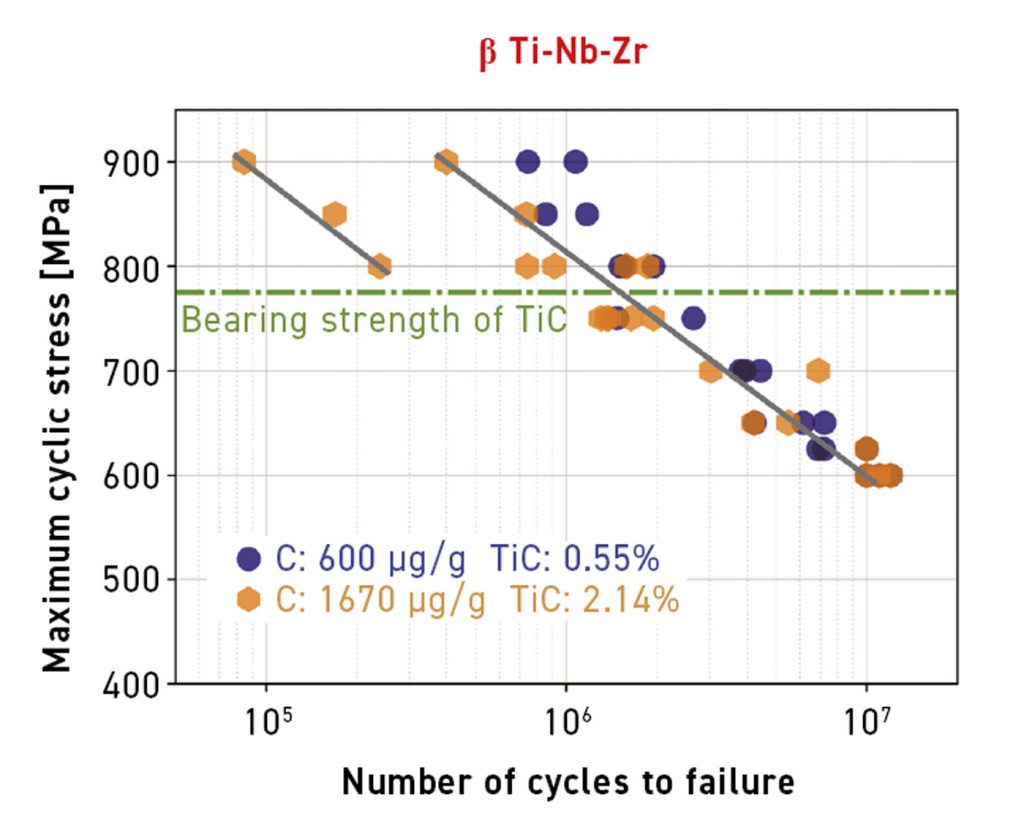
In summary, the study discovered a rather complex mechanism of crack initiation consisting of an interplay of pores, fishbone-like alpha platelets at grain boundaries and titanium carbides. However, with respect to grain size and impurity, the fatigue behaviour of β-titanium alloys appears to be more robust than α/β alloys. Fig. 22 shows the measured fatigue curve in comparison of Ti-6Al-4V and Ti-6Al-7Nb and Ti-20Nb-10Zr. Although grain size and oxygen content were much higher, the Ti-20Nb-10Zr alloy shows a significantly higher fatigue resistance.
![Fig. 22 Fatigue behaviour of Ti-20Nb-10Zr in comparison to Ti-6Al-4V and Ti-6Al-7Nb. All samples were fabricated by MIM [17]](http://www.pim-international.com/wp-content/uploads/sites/2/2023/08/Fig-22-1024x824.jpg)
The potential of eutectoid β stabilising elements to develop new PMTi alloy compositions
At earlier PMTi meetings, Leandro Bolzoni from the University of Waikato, New Zealand, presented studies on low-cost α/β-titanium alloys. In Montréal, he reported on an investigation on alloy systems of the form Ti-2X-2Z, where ‘X’ means an isomorphous β-stabilising alloying element (e.g., Nb) and ‘Z’, a eutectoid one (e.g., Cu, Mn or Fe) [18]. In doing so, Bolzoni tried to combine both effects in order to minimise the necessary amount of alloying elements – and the rather expensive Nb in particular.
As before, Bolzoni applied the blended elemental approach, mixed the powders in the desired ratio, compacted them by uniaxial pressing with 600 MPa and finally sintered under vacuum at 1300°C for 2 h with subsequent furnace cooling. He characterised all specimens by XRD and microscopy, as well as hardness and tensile testing, and determined density, porosity and densification. Alloy compositions of Ti-2Nb-2Cu, Ti-2Nb-2Mn, Ti-2Nb-2Fe, Ti-2Fe-2Cu and Ti-2Fe-2Mn were chosen and compared with unalloyed Ti, with powder shape ranging from spherical to irregular.
Bolzoni observed some general rules related to the alloying elements and their effect on densification: Generally, Nb hinders densification, while Fe and Mn favour it. Moreover, within the Nb-based alloys, Cu shows the lowest positive effect on densification and Fe the highest. Within the Fe-based alloys, Nb revealed the weakest effect and Mn the strongest. In all cases, XRD detected no intermetallic phases. In addition, Cu-bearing alloys revealed no β-phase by XRD, though this was visible in the micrographs as part of a lamellar α/β structure. Generally, in Nb-based alloys, the lamellae were refined by the additional alloying elements in the order Cu->Fe->Mn, while in Fe-based types the order of effect was Cu->Nb->Mn. Bolzoni emphasised that the classification of the alloys according to the Molybdenum-Equivalent approach did not match the microstructural results in all cases, and that this theory needs more specificity to be able to take into account the effect of the processing conditions (i.e., cooling rate). Mostly, the alloys of the Ti-2X-2Z type can be classified as near-α or α/β alloys. The mechanical properties tend to show higher strength and ductility compared to cast Ti-xCu and wrought Ti-xNb alloys. This is probably due to the finer microstructure of the Ti-2X-2Z alloys and the strengthening effect of the additional alloying elements.
The evolution of automotive applications for powder-based titanium processes
A very comprehensive talk on development of Ti alloys was given by Tadahiko Furuta from Toyota Central R&D Labs. Inc. (TCRDL), Japan, focusing on the alloys’ application in the automobile sector [19]. TCRDL has a long history in this field; indeed, they already use special Ti materials for motor valves in passenger cars and motorbikes. Here, high performance in combination with low costs is essential, and the approach of using blended elemental powders to fabricate TiMMCs (Metal Matrix Composites) was successfully followed.
Motor valves require a high Young’s modulus of the material, high wear resistance, as well as excellent fatigue and creep properties at elevated temperatures. Furuta described the development process, starting with the choice of reinforcement particles. From a few different materials like TiC, TiN, SiC, B4C and others, he selected TiB as the best candidate because of its high Young’s modulus and hardness, a coefficient of linear expansion close to that of titanium and excellent stability in Ti alloys. Fabrication started with blending TiH2 and 16% of Al-25Sn-25Zr-6Nb-6Mo-1.2Si, resulting in the alloy Ti-6Al-4Sn-4Zr-1Nb-1Mo-0.2Si-0.3O during sintering. TiB2 powder was added to this mixture, compacted and vacuum sintered at 1300°C. During this process TiH2 and TiB2 react, forming TiB particles. The sintered billets were hot worked by extrusion and forging and final upsetting to shape the valve. Subsequent heat treatment, machining and coating led to the finished product. A further variant of development was the alloy Ti-6Al-4V-2Mo-1Fe, reinforced by 20 vol.% TiB.
TEM examinations revealed the excellent atomic bonding between the beta phase and the TiB particle, resulting in high mechanical properties at elevated temperatures. Fig. 23 shows the general improvement of the fatigue properties of the developed βTiMMCs compared to Ti-6Al-4V. The mechanical properties of these alloys can be adjusted by heat treatments and the number of reinforcing particles. Furuta showed how the properties can be fine-tuned to the demands from application and production (e.g., with respect to durability and productivity).
![Fig. 23 Comparison of mechanical properties of Ti-6Al-4V and Ti-MMCs reinforced with TiB [19]](http://www.pim-international.com/wp-content/uploads/sites/2/2023/08/Fig-23-1024x653.jpg)
Despite the excellent performance and low cost production approach for this type of TiMMCs, however, costs are still too high to make sense as a regular material for passenger vehicles.
Furuta also presented a second titanium alloy called Gum Metal. The composition is based on the effect of dependence of the Young’s modulus on the number of alloying elements – here, of Ta, V and Nb. A minimum of this value exists at 25 mol.%, which is found experimentally, but can also be calculated based on electron theory. The lowest value of the Young’s modulus is achieved in the case of Nb, but adding further elements can increase this effect. The purpose of Gum Metal’s creation was to develop a high-strength alloy with a low Young’s modulus. As production method, the same blended elemental approach was used as for the TiMMCs, though with an additional cold working step after hot working.
After cold working, a non-linear elastic behaviour could be observed; this led to a high elastic strain like 2.5% in the case of a Ti-23Nb-0.7Ta-2.0Zr-1.2O alloy. This alloy has a YS around 1000 MPa. Furuta showed that the amount of oxygen is essential to control this elastic behaviour: if the O content is too low, strain is high, but strength is very low. If the concentration of oxygen is too high, linear elastic behaviour is visible. High-resolution TEM investigations revealed that recoverable localised shear deformation is the basic underlying mechanism which is highly dependent on the number of oxygen atoms. The effective deformation ranges from nanometres to millimetres in Gum Metal. Application of this alloy span from highly flexible spectacle frames, screws and bolts with anti-loosening effect to orthodontics.
Furuta closed his talk with some general remarks about new titanium alloys for the automobile sector, including those which feature a level of electrical resistance in order to reduce eddy current losses. He summarised the possible approaches for alloy development as shown in Fig. 24. His talk illustrated how important it is to connect experimental evidence with theoretical knowledge.
![Fig. 24 General concepts for the development of novel high-strength Ti alloys [19]](http://www.pim-international.com/wp-content/uploads/sites/2/2023/08/Fig-24-1024x935.jpg)
Development of high-strength Ti alloys based on the composite approach
The development of high-strength titanium alloys based on the composite approach was also presented by Yong Liu from Central South University in Changsha, China [20]. In the beginning of his presentation, he pointed out one disadvantage of common MMCs using ceramic particles as reinforcement: common loss in ductility and risk of bonding failure between ceramics and metal matrix. In contrast, Liu’s approach was influenced by the well-known Damascus steel, which consists of a hard and a soft metal phase kneaded together and results in excellent strength and ductility.
Thus, the idea presented by Liu was to focus on strengthening via a heterogeneous structural mixture of at least two metallic phases with a high density of interfaces. To generate such structures, any possible diffusion of the alloying elements must be kept low to avoid homogenisation. Therefore, Liu chiefly applied methods like SPS and hot working, thereby minimising the processing temperature.
One example he gave was the fabrication of a Ti-Ta composite using the blended elemental approach. Because Ta diffuses slowly, only 2 µm powder was used. The powder mixture was processed by SPS at 1200°C for 30 min, hot rolled at 700°C and finally annealed at 600°C for 30 min. The result was a very fine (< 100 nm) laminate-structured Ta-rich zone with nanoscale precipitates in the Ti-rich zone. In addition, slight diffusion occurred between these two zones. After annealing, the UTS achieved was 1210 MPa and elongation to fracture was 20% – excellent properties.
A second example presented by Liu was a TiMo composite fabricated by blending Ti, 40Al60V and Mo powders, Cold Isostatic Pressing (CIP) at 200 MPa, sintering at 1200°C for 2 h and final hot swaging at 950°C. The result was a compositional gradient in the microstructure, causing inhomogeneous precipitation of α-phase as visible in Fig. 25. The Mo-enriched zone consists of β-phase, the Ti-enriched zones feature α and β. The resulting strength of this composite was very high, with a UTS of 1634 MPa. The elongation was 8%, which is a an acceptable value for most applications and significantly better than for alloys with comparable strength.
![Fig. 25 Compositional and resulting structural gradient in Ti-Mo composite [20]](http://www.pim-international.com/wp-content/uploads/sites/2/2023/08/Fig-25-1024x748.jpg)
In the second half of his talk, Liu discussed the underlying physical mechanisms for strengthening based on the example of the Ti-Ta composites. It is quite obvious that a combination of strengthening factors such as the solid solution, grain boundary, dislocation and precipitation is responsible for the high UTS and YS values. However, when Liu applied the classical strengthening models, summing up all single contributions, the calculated YS value was about 200 MPa lower than the measured one. Liu suggested this missing contribution to be attributed to the Heterogeneous-Deformation-Induced (HDI) strengthening effect. This effect results from the interface between the soft Ta and the hard Ti-enriched phases.
After a tension load is applied, the dislocation density in the Ta-rich zone is higher than in the Ti-rich zone. This effect is even enhanced by the rolling process introducing more additional dislocations in the Ta-rich regions due to the higher strain gradient caused by existing nano-precipitates. Interestingly, as an in-situ tensile test on nano-machined pillars of the different zones showed, the soft Ta-rich zone is strengthened by the HDI effect to values similar to the strength of the Ti-rich zone.
Liu tried to calculate the HDI induced strength on the basis of a traditional back stress model, but failed; the calculated value was much too high compared to the 200 MPa difference assessed experimentally. Thus, he developed a new model considering the residual plastic strain energy. As experimental input, the result of a loading – unloading – loop tensile test is suitable. With this updated model, the results of the experimentation can be visualised in Fig. 26.
![Fig. 26 Comparison of experimental result, the traditional and a newly developed model for the HDI contribution of strengthening in Ti-W composite [20]](http://www.pim-international.com/wp-content/uploads/sites/2/2023/08/Fig-26-1024x816.jpg)
Conclusion
To conclude, the PMTi2022 conference proved that there is still high interest in titanium – and as many questions remain open as ever. Will viable novel solutions become cost effective? Will the importance of alloy development grow beyond thermomechanical processing? Will standards hinder the development of application-specific AM alloys?
Regarding that last point, there was an interesting podium discussion organised during the conference on the question of whether it is actually necessary to fulfil the chemical requirements of standards if the mechanical ones are achieved perfectly well – in particular, this is an issue with oxygen content. There was no clear result of the discussion – too many aspects have to be considered – but this discussion could be the start of a fundamental change in standards.
To date, many different manufacturing techniques exist, and the processing of the same raw material can lead to identical high mechanical properties even if microstructure and impurity content differ. Should there actually be a standard for each technique, or is there a different way of handling this situation? Maybe this discussion will be concluded at PMTi2023 – or maybe not. Attend the conference at the University Carlos III in Madrid, Spain, in the first week of September to be part of the conversation!
Author
Dr Thomas Ebel
Helmholtz-Zentrum Hereon
Powder-Based Materials Development
Institute of Metallic Biomaterials
Geesthacht
Germany
[email protected]
www.hereon.de
References
[1] Zhigang Zak Fang, A Holistic approach to Low-Cost Titanium
[2] Muktesh Paliwal et al., HDH Ti-6Al-4V Alloy for Laser Powder Bed Fusion
[3] Gang Chen et al., Cost Down and Lean Design of High- Performance CP-Ti Via Additive Manufacturing
[4] Chengshang Zhou et al., Improving Densities of Titanium Alloys Using Constrained Hydrogenation Assisted Densification (CHAD) Method
[5] Efraín Carreño-Morelli, Powder Metallurgy of Titanium-Nickel Alloys: An Overview
[6] Paul Sheffield et al., Evaluation of Nitinol Produced via Multiple PM Forming Routes
[7] Carlos Romero et al., Opportunities of Powder Metallurgy Titanium in the Hydrogen Technologies: The Case of Bipolar Plates
[8] Robert Swenson, Titanium Metal Injection Molding and 3D Binderjet Printing
[9] Christian Doblin et al., Comparative assessment of wrought & PM Ti-6242 (Ti-6Al-2Sn- 4Zr-2Mo-0.8Si) alloy rods fabricated using cold spray, hot isostatic pressing, and spark plasma sintering
[10] Alex Finch, Cold Spray Additive Manufacturing: Breakthrough Performance and Economics in Titanium Additive Manufacturing
[11] Shannon Clemens, Examination of Cold Rolling and Annealing Processes for Ti-6Al-4V and Ti-5Al-2.5Fe Roll Compacted Strips
[12] Matthias Scharvogel, Scaling Sinter Based 3D Printing Technologies for Titanium and Its Alloys
[13] Johannes Schaper, Sinter Based Additive Manufacturing of Titanium, Binder, and Processing Technology
[14] Wolfgang Limberg et al., Comparison of Fatigue Properties of Ti- 6Al-4V, Processed by Metal Injection Molding and Fused Granular Fabrication
[15] Salah Eddine Brika et al., A Novel Testing Bench for the Assessment of Metal Powder Spreading Behavior for Powder Bed-Based AM Applications
[16] Karin Ratschbacher, Production Routes for Specialized, Spherical, Biocompatible Ti-Based Powders
[17] Thomas Ebel, Fatigue Behavior and Failure Mechanisms of Metal- Injection-Molded Beta Titanium Alloys
[18] Leandro Bolzoni, On the potential of Eutectoid β Stabilizing Elements to Develop New PM Ti Alloy Compositions
[19] Tadahiko Furuta, Titanium Products via Powder Metallurgy Process
[20] Yong Liu et al., Mechanical Behavior of Heterogeneous Ti Metal-Metal Composites